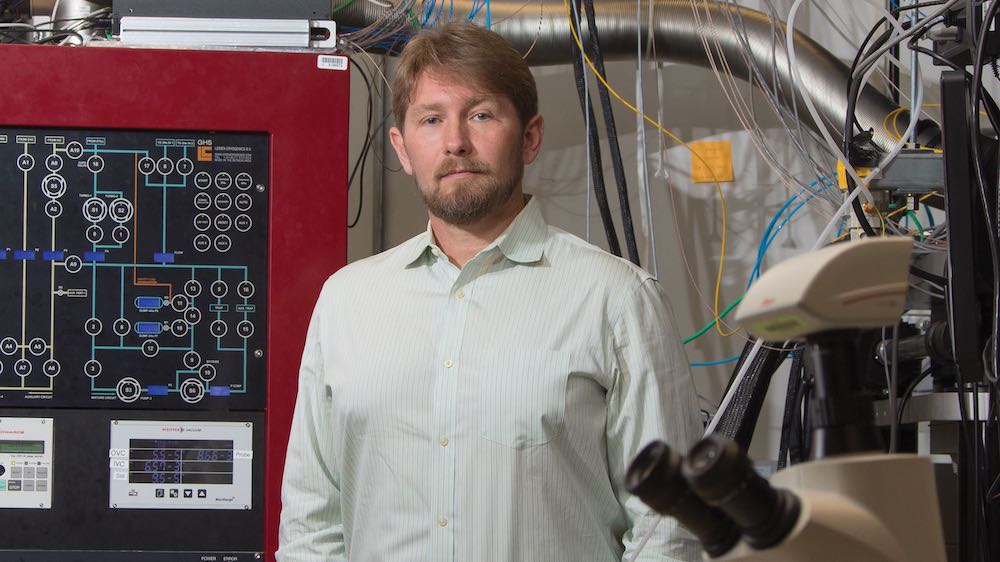
ORNL quantum computing scientist Travis Humble. Image credit: Carlos Jones, ORNL
Building on qubits
The basic unit of computing on a quantum computer is known as a qubit (short for “quantum bit” and pronounced CUE-bit). A qubit is comparable to the bits that govern your day-to-day computer, but there are important differences owing to the quantum mechanical nature of qubits.
For example, a bit (short for “binary digit”) must be one of two values, usually stated as 0 and 1. But qubits, because they are quantum mechanical, don’t have that restriction. The quantum principle of superposition means a qubit can be a 0, a 1, or some combination of both. As a result, a qubit can represent any one of an essentially limitless range of possible values.
In addition, qubits are subject to quantum entanglement, a special case of superposition in which the two-qubit state is well defined but neither individual state has definite properties. This allows qubits and other quantum particles to establish strong quantum correlations over long distances.
Quantum computers for quantum problems
Researchers have been intrigued by the possibility of quantum computing at least since the early 1980s, going back to the colorful Caltech physicist Richard Feynman’s declaration that “nature isn’t classical, dammit, and if you want to make a simulation of nature, you’d better make it quantum mechanical.”
Nearly 40 years later, experts agree that the quantum world of molecules, atoms and electrons does indeed present an especially fertile ground for this evolving technology.
“A quantum computer is really good at solving quantum mechanics problems,” explained ORNL quantum computing scientist Travis Humble.
“So finding what an electron in a molecule is doing—quantum computers are good at that. Looking at the electronic structure of the molecule—the different low-energy levels it has—that information is what we use in chemical reactions.”
These problems are especially challenging for classical computers because quantum systems are, by their nature, uncertain. If an electron were a classical particle, for instance, its internal magnet—or spin—would point either up or down, and all you needed to know about that spin could be recorded in a single bit’s value of 0 or 1.
But that electron is not classical, it’s quantum, meaning its spin can be up, down, or a combination of both. So, to even begin simulating the complexity of a quantum system, a classical computer must be very powerful and have a vast amount of memory.
Qubits, on the other hand, can more easily reflect the nature of a quantum system because qubits are themselves uncertain.
“Exponential growth is naturally accounted for if you’re using quantum systems to simulate quantum systems,” explained ORNL quantum information researcher Pavel Lougovski. “You don’t have to parse it into classical bits.”
Humble noted that quantum computing is also promising in areas like computational chemistry, where the correlated behavior of an atom’s nucleus and its electrons determines the stability of a molecule. As an example, he noted that advances in quantum computing may enable scientists to discover more efficient methods to synthesize novel chemicals.
“Finding energy-efficient pathways for molecular synthesis is a hard computational problem,” Humble explained. “There are so many different ways for atoms to bond together, and the best configurations often arise from unintuitive quantum mechanical effects. But quantum computing offers a new approach to simulate how molecules react under different conditions, and we expect this to have a significant impact on computational chemistry.”
The basic unit of computing on a quantum computer is known as a qubit (short for “quantum bit” and pronounced CUE-bit). A qubit is comparable to the bits that govern your day-to-day computer, but there are important differences owing to the quantum mechanical nature of qubits.
For example, a bit (short for “binary digit”) must be one of two values, usually stated as 0 and 1. But qubits, because they are quantum mechanical, don’t have that restriction. The quantum principle of superposition means a qubit can be a 0, a 1, or some combination of both. As a result, a qubit can represent any one of an essentially limitless range of possible values.
In addition, qubits are subject to quantum entanglement, a special case of superposition in which the two-qubit state is well defined but neither individual state has definite properties. This allows qubits and other quantum particles to establish strong quantum correlations over long distances.
Quantum computers for quantum problems
Researchers have been intrigued by the possibility of quantum computing at least since the early 1980s, going back to the colorful Caltech physicist Richard Feynman’s declaration that “nature isn’t classical, dammit, and if you want to make a simulation of nature, you’d better make it quantum mechanical.”
Nearly 40 years later, experts agree that the quantum world of molecules, atoms and electrons does indeed present an especially fertile ground for this evolving technology.
“A quantum computer is really good at solving quantum mechanics problems,” explained ORNL quantum computing scientist Travis Humble.
“So finding what an electron in a molecule is doing—quantum computers are good at that. Looking at the electronic structure of the molecule—the different low-energy levels it has—that information is what we use in chemical reactions.”
These problems are especially challenging for classical computers because quantum systems are, by their nature, uncertain. If an electron were a classical particle, for instance, its internal magnet—or spin—would point either up or down, and all you needed to know about that spin could be recorded in a single bit’s value of 0 or 1.
But that electron is not classical, it’s quantum, meaning its spin can be up, down, or a combination of both. So, to even begin simulating the complexity of a quantum system, a classical computer must be very powerful and have a vast amount of memory.
Qubits, on the other hand, can more easily reflect the nature of a quantum system because qubits are themselves uncertain.
“Exponential growth is naturally accounted for if you’re using quantum systems to simulate quantum systems,” explained ORNL quantum information researcher Pavel Lougovski. “You don’t have to parse it into classical bits.”
Humble noted that quantum computing is also promising in areas like computational chemistry, where the correlated behavior of an atom’s nucleus and its electrons determines the stability of a molecule. As an example, he noted that advances in quantum computing may enable scientists to discover more efficient methods to synthesize novel chemicals.
“Finding energy-efficient pathways for molecular synthesis is a hard computational problem,” Humble explained. “There are so many different ways for atoms to bond together, and the best configurations often arise from unintuitive quantum mechanical effects. But quantum computing offers a new approach to simulate how molecules react under different conditions, and we expect this to have a significant impact on computational chemistry.”
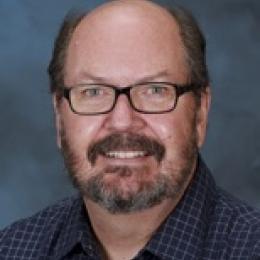
Leo L Williams, Editor, ORNL Review
A long way to go
As promising as quantum computing appears, many hurdles must be cleared before it provides important scientific discoveries. There are working quantum computers, created by tech giants such as Google, IBM and Intel as well as smaller companies such as British Columbia-based D-Wave. Yet so far these rudimentary systems serve more to advance research into quantum computing than to provide new scientific knowledge.
“The hardware that is available on the cloud, for example, has numbers of qubits that are on a par with what we can simulate classically,” Lougovski said, “so there is, per se, no new science to discover, except for the science of computing—such as how we program those devices, and how we find ways to extract errors and improve the results.”
“The rudimentary processors we have right now are basically physics experiments,” agreed Humble. “They are almost always located in a laboratory. They do have ways to interface with some—write programs, collect answers and solutions. But it’s very finicky."
“So the question is, what do they need to get up to before they are ready for the Department of Energy, for example, to say, ‘I’m going to build a supercomputing system based on quantum processors.’”
Looking for the right qubit
A qubit can be any particle or system that exhibits superposition and quantum entanglement. To date, the field is dominated by qubits consisting of superconducting circuits, yet researchers are studying a range of possibilities in the hope that one or more will be stable enough to make quantum computing practical.
These possibilities include tiny specks of silicon called silicon quantum dots; trapped ions, in which tiny charged particles are held in place by electromagnetic fields; and topological insulators, or materials in which electrons in the center are held in place while electrons at the edge are allowed to form a circuit.
“One major goal is to find materials systems that allow qubits to persist for long periods of time," explained ORNL materials scientist Stephen Jesse. “This may happen at particular defect sites in an otherwise perfect materials system that are inherently immune to external perturbations, or this could be in materials systems where the quantum state is ‘protected.’”
Bring in the noise
The search for a more stable qubit is critical because of quantum particles’ enormous sensitivity to their environments. While this makes them very promising candidates for advanced sensors, it is a serious challenge for the development of useful quantum computers. Not only must quantum computers harness the delicate states of electrons and other tiny particles, they must also prevent those particles from being disturbed by events such as vibrations, electrical fields and radiant heat.
In fact, Jesse noted, quantum computers must be kept very close to absolute zero, or below minus-450 degrees Fahrenheit.
“These qubits are very sensitive to everything that happens around them. They usually have to run these computers at 20 millikelvin, just barely above Kelvin zero, because any kind of disturbance can knock these guys out of states that they need to be in.”
Even something as seemingly insignificant as energy emanating from a wire running into a quantum computer can render it essentially useless, explained ORNL Quantum Communications Team lead Nicholas Peters.
“For most qubits, it’s expected that we are going to end up having to have them be very, very cold,” Peters said. “Just having a wire at room temperature gives you enough noise from the blackbody emission that it will basically ruin your ability to use those as qubits.”
In other words, quantum computer developers must figure out how to control and read the processors while at the same time keeping them frigid.
“These are two competing processes,” Lougovski said. “You would like to isolate your qubits from the environment so that they are not affected by noise, but on the other hand, you want to be able to send control and readout pulses to those systems to get the information in and out.”
According to Peters, this means either generating the signal in this frigid environment or, more commonly, building an interface that carries the signal without carrying the heat.
“At each of these stages, what you end up doing is throwing a whole bunch of attenuators to block the blackbody radiation from higher up,” Peters explained. “Then you also have to heatsink it to try to cool that wire down so that it doesn’t shine its own blackbody radiation down further into the fridge.”
A question of decoherence
These efforts are geared to keeping the qubits from collapsing—or losing their quantum states—long enough to perform useful calculations. So far, this challenge remains daunting.
While current systems can—in theory—perform around a thousand operations before they lose their information, Humble said, the number in practice is dramatically lower—around two operations. As a result, any problem that can be tackled on a quantum computer can also be tackled on a classical computer.
“Going back to the technical challenges, it’s that noise. It is really an engineering problem at the moment. Lowering the noise to a level where you could get a hundredfold improvement is where the challenge is.”
As promising as quantum computing appears, many hurdles must be cleared before it provides important scientific discoveries. There are working quantum computers, created by tech giants such as Google, IBM and Intel as well as smaller companies such as British Columbia-based D-Wave. Yet so far these rudimentary systems serve more to advance research into quantum computing than to provide new scientific knowledge.
“The hardware that is available on the cloud, for example, has numbers of qubits that are on a par with what we can simulate classically,” Lougovski said, “so there is, per se, no new science to discover, except for the science of computing—such as how we program those devices, and how we find ways to extract errors and improve the results.”
“The rudimentary processors we have right now are basically physics experiments,” agreed Humble. “They are almost always located in a laboratory. They do have ways to interface with some—write programs, collect answers and solutions. But it’s very finicky."
“So the question is, what do they need to get up to before they are ready for the Department of Energy, for example, to say, ‘I’m going to build a supercomputing system based on quantum processors.’”
Looking for the right qubit
A qubit can be any particle or system that exhibits superposition and quantum entanglement. To date, the field is dominated by qubits consisting of superconducting circuits, yet researchers are studying a range of possibilities in the hope that one or more will be stable enough to make quantum computing practical.
These possibilities include tiny specks of silicon called silicon quantum dots; trapped ions, in which tiny charged particles are held in place by electromagnetic fields; and topological insulators, or materials in which electrons in the center are held in place while electrons at the edge are allowed to form a circuit.
“One major goal is to find materials systems that allow qubits to persist for long periods of time," explained ORNL materials scientist Stephen Jesse. “This may happen at particular defect sites in an otherwise perfect materials system that are inherently immune to external perturbations, or this could be in materials systems where the quantum state is ‘protected.’”
Bring in the noise
The search for a more stable qubit is critical because of quantum particles’ enormous sensitivity to their environments. While this makes them very promising candidates for advanced sensors, it is a serious challenge for the development of useful quantum computers. Not only must quantum computers harness the delicate states of electrons and other tiny particles, they must also prevent those particles from being disturbed by events such as vibrations, electrical fields and radiant heat.
In fact, Jesse noted, quantum computers must be kept very close to absolute zero, or below minus-450 degrees Fahrenheit.
“These qubits are very sensitive to everything that happens around them. They usually have to run these computers at 20 millikelvin, just barely above Kelvin zero, because any kind of disturbance can knock these guys out of states that they need to be in.”
Even something as seemingly insignificant as energy emanating from a wire running into a quantum computer can render it essentially useless, explained ORNL Quantum Communications Team lead Nicholas Peters.
“For most qubits, it’s expected that we are going to end up having to have them be very, very cold,” Peters said. “Just having a wire at room temperature gives you enough noise from the blackbody emission that it will basically ruin your ability to use those as qubits.”
In other words, quantum computer developers must figure out how to control and read the processors while at the same time keeping them frigid.
“These are two competing processes,” Lougovski said. “You would like to isolate your qubits from the environment so that they are not affected by noise, but on the other hand, you want to be able to send control and readout pulses to those systems to get the information in and out.”
According to Peters, this means either generating the signal in this frigid environment or, more commonly, building an interface that carries the signal without carrying the heat.
“At each of these stages, what you end up doing is throwing a whole bunch of attenuators to block the blackbody radiation from higher up,” Peters explained. “Then you also have to heatsink it to try to cool that wire down so that it doesn’t shine its own blackbody radiation down further into the fridge.”
A question of decoherence
These efforts are geared to keeping the qubits from collapsing—or losing their quantum states—long enough to perform useful calculations. So far, this challenge remains daunting.
While current systems can—in theory—perform around a thousand operations before they lose their information, Humble said, the number in practice is dramatically lower—around two operations. As a result, any problem that can be tackled on a quantum computer can also be tackled on a classical computer.
“Going back to the technical challenges, it’s that noise. It is really an engineering problem at the moment. Lowering the noise to a level where you could get a hundredfold improvement is where the challenge is.”